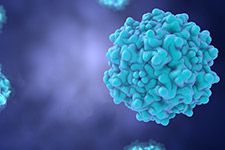
By Feliza Mirasol
Viral vectors show promise as a delivery mechanism for gene therapy, but which virus types are commercially viable?
Several virus types have been studied for use as viral vectors in gene therapy, including adenovirus, lentivirus, retrovirus, and adeno-associated virus (AAV). Of these, AAVs have gained much attention as a gene delivery vehicle and are being explored for their commerical viability. The commercial viability of other virus types also continues to be explored, particularly for treatments that require a larger payload, transient expression, or insertion of a gene of interest in the genome.
The potential opportunity of a vector depends on the type of gene therapy being developed, says Gaurav Chaudhary, CEO of Roots Analysis, a business research and consulting firm. In general, there are two types of gene therapies, namely ex-vivo and in-vivo, Chaudhary points out. “In ex-vivo, cells are modified outside the patient’s body and the corrected version is transplanted back into the patient. Opposite of ex-vivo is what we call in-vivo, where cells are treated inside the patient’s body. The corrected copy of the genes is transferred into the body of the patient.”
In both cases, cells may be treated either with a viral or non-viral vector carrying the corrected copy of the gene. Generally, AAV and adenoviruses are primarily used for creating in-vivo gene therapy. Compared to adenovirus and AAV, retrovirus-based vectors show relatively low transduction efficiency in-vivo, but their advantage lies in their ability to integrate stably and confer long-term transgene expression in dividing tissues. Retroviruses and lentiviruses, therefore, are most suitable for ex-vivo gene transfer, Chaudhary states.
Each viral vector system is characterized by an inherent set of properties that affect its suitability for gene therapy or other specific applications, says Florence Vicaire, global gene therapy business development leader, Cytiva. “There is no one-fits-all multipurpose viral vector appropriate for all applications; each of the vectors has its own advantages, limitations, and range of applications,” Vicaire says.
The viral-vector mechanism
Viral vector systems are gutted viruses where the packaging signals (e.g., the genetic signals that target the wrapping into the viral particles) are fused to the genes of interest (the payload), and the remaining necessary elements are placed on separate genetic elements, often three or more, says Carsten Carstens, senior scientist, R&D, Agilent Technologies.
“The objective here is to prevent formation of a replication-competent viral particle,” Carstens emphasizes. “The non-payload elements are generally referred to as the packaging system. If performed properly, packaging results only in infectious particles carrying the payload.”
The primary objective of these viral vector systems is to achieve the highest possible titers without forming replication-competent variants. “For lentiviral packaging, there are iterative cycles of safety improvements. Agilent vectors, for example, are suitable and compatible with generation-four packaging systems (i.e., the latest version of packaging systems).”
For all practical purposes, a viral vector is a genetic element wrapped in its own transfection reagent, which makes transfection titrations possible (e.g., dosage responses) and highly effective. “In an R&D setting, this becomes preferable if the same reagent is transfected (for example, CAS9 delivery vectors) since all the work is in the generation of the viral stock, not in the infection,” Carstens says.
Carstens points out that the greatest limitation of viral vector systems is their tropism, that is, the type of cells that a virus will naturally infect. Infectivity, he explains, is usually dictated by the envelope proteins present in a virus and the cellular surface components to which those proteins bind. “Depending on the application, the ideal vector either has an extremely broad tropism or extremely narrow if the objective is gene therapy of a very specific cell type (in this case this is a safety precaution),” Carstens elucidates.
Some vectors, such as adenoviruses, have a fairly broad tropism, and the target range is often modified by engineering part of the envelope proteins. Carstens cautions, however, that biomanufacturers need to be aware that such modifications are properties of the packaging system, not the viral vector. “The process of changing the tropism of the vectors is referred to as pseudotyping. For instance, Agilent has retroviral and lentiviral vector systems that are pseudotyped to achieve a wide target range. The upside of pseudotyping is a tailored target range. The downside is that some modifications of the envelopes lead to destabilization of the viral particle and that pseudotyping requires engineering of the packaging system that is often not accessible to most customers,” Carstens states.
Lentiviral vectors, meanwhile, are a subgroup of retroviral vectors. Lentiviral vectors have displaced traditional retroviral vectors because lentiviral vectors do not require dividing host cells for integration, Carstens further explains. This characteristic broadens the utility of lentiviral vectors compared to traditional retroviral systems. Upsides to using a lentiviral vector include the ability of the system to carry a relatively large payload and genetic elements that become active only after integration. This makes it relatively straightforward to achieve desired titers and to achieve a single integration element, Carstens emphasizes. Downsides include the fact that it is more difficult to achieve large titers. In addition, each integration event constitutes a mutation in its own right, which becomes a safety concern when it comes to gene therapy, Carstens notes.
In comparison, adenoviral vectors do not integrate into the host genome and are thus a transient delivery system. “They were once thought to have potential in gene therapy approaches, but there are potentially strong immune responses when used in vivo, which is why they are typically not used in gene therapy approaches anymore,” Carstens states.
Finally, AAV is a “defective” virus that is not replication competent by itself. “[AAV] is famous as being the only known vertebrate virus to form a lysogen, meaning it integrates into the genome in a dormant state from which it can be rescued by superinfection with certain viruses, most notably the adenovirus (hence its name) or by some forms of chemical treatments (butyric acid and others),” notes Carstens. The natural defectiveness of AAV makes it an inherently safe delivery system.
Most packaging systems will result in infectious particles that will not, or at least infrequently, integrate into the genome, and, thus, infection results in transient expression such as the adenoviral vectors. “The virus can integrate into the genome at a very specific site (e.g., the ‘lysogenic’ form). However, this requires modification to the packaging system,” says Carstens. Upsides to AAV include the relative safety of these vectors (versus lentiviral), relatively low immune responses, and reasonably broad tropism. Some of the downsides include a limited payload, difficulty achieving high titers and functional titers, and difficulty in pseudotyping, Carstens reports.
The promise of AAV
Of the different virus types that have been explored for gene therapy, AAV is the most commonly used because it offers certain advantages that make it a promising vector compared to other vector types. Chaudhary points out some of the advantages that AAV offers:
- AAV possesses characteristics that allow efficient manipulation of the vector (as required).
- AAV possesses the ability to be easily purified, as they are not readily degraded by shear forces, enzymes, or solvents.
- AAV exhibits reduced risk of adverse inflammatory reactions because of its non-pathogenic nature and because it has less immunogenic properties.
- AAV allows for the delivery of genetic sequences of up to approximately 4 kb.
- AAV exhibits reduced risk of ectopic integration of the therapeutic DNA.
“Adenovirus (AV) was the first viral vector investigated for gene therapy use; however, after the death of a patient in 1999 due to immunogenic reaction against AV (1), researches on gene therapy using AV were put to a halt, and other vectors were investigated, including AAV, especially due to AAV’s lower immunogenicity. The latest approvals of gene therapies using AAV (e.g., Luxturna, Zolgensma) have led to a surge in AAV use as it has proven safe and effective,” adds Vicaire.
Based on recent approvals and the benefits of AAV, including its low immunogenicity, the fact that it does not cause any disease in humans—something other viral vectors have the potential to do—its ability to confer long-term gene expression in vivo, its potential to infect replicative as well as non-replicative cells, and its potential to transduce a wide range of tissues, AAV is currently considered the best viral vector for in-vivo gene therapy, and is also considered as a potential delivery vehicle for other applications, such as gene editing, asserts Vicaire.
By now, a wide variety of AAV serotypes have been studied, adds Kai Touw, technical lead at Batavia Biosciences, a Netherlands-based contract development and manufacturing organization (CDMO). Some of the serotypes have been successful preclinical and clinical followed by regulatory approval in both Europe and the United States. “This makes it a popular starting point for gene therapy, although many serotypes continue to struggle with manufacturing challenges, such as low production yields and therefore high cost of goods,” Touw says.
“Two distinct advantages of AAV vectors is the safety profile at large doses and the long-term expression (durability) of a gene of interest. This means they can be used to treat genetic disorders, such as spinal muscular atrophy and hemophilia,” Chris Murphy, vice-president and general manager, Viral Vector Services, Thermo Fisher Scientific, further adds.
“Most packaging systems with AAV have been engineered so that it does not integrate into the host genome, and, as a result minimizes any potential non-intended mutations, versus lentiviral vectors, which are only active after integration. The relatively low immune response compared with adenoviral vectors makes AAV a more favorable approach,” concurs David Weiss, product manager, Genome Engineering, Agilent Technologies.
Manufacturing bottleneck
Manufacturers, meanwhile, are contending with the challenges of manufacturing viral vectors. Among the main manufacturing challenges today for AAV serotypes is the use of the adherent human embryonic kidney-293 (HEK-293) cell line. The current AAV manufacturing process using the HEK-293 cell line can only be increased by a “scale-out” method, notes Touw. This is carried out by implementing more hyperstacks, or cell stacks, for production. “Scale-out of these systems result in more laboratory handlings and, subsequently, a higher failure rate due to contaminations,” Touw says.
“This can be prevented by implementing a novel fixed-bed bioreactor system for adherent cell production like the iCELLis (Pall) or scale-X system (Univercells),” Touw continues. “Batavia managed to collaborate with the suppliers to optimize these systems and make them more suitable for process development and clinical manufacturing, specifically for viral vectors, leading to lower failure rates from significantly less contaminations and, therefore, lower cost of goods.”
With a better understanding of the upstream process for the various AAV vectors, productivity can be increased, but this could give rise to new challenges downstream, such as changes in the impurity profile of the cell harvest, Touw adds. “Because each serotype has its own challenges, it’s not possible to simply add in available plug-and-play technologies,” he states.
More recent innovations, such as novel membranes and monoliths, are suitable as a capture step, Touw notes, while at the same time lowering buffer consumptions. Other innovations, such as affinity ligands for specific AAV serotypes are being developed for use with these technologies to further enhance the resolution of the capture step. A major challenge remains, however, and that is to control the amount of empty and hence non-infectious particles during manufacturing.
“For this, we also should not forget Industry 4.0 and the use of data analytics and machine learning within biopharma (such as multivariate data analysis). This will influence the production of AAV and other viral vectors. Learning how process parameters in the upstream process influence the purification process is fundamental for a good quality-by-design strategy. Furthermore, it will be interesting to see the developments in the intensification of monoclonal antibody (mAb) production processes. Lessons learned and technologies developed here could have the same benefits for the production of viral vectors,” says Touw.
“After production, vectors need to be recovered from large volumes of cell lysate or medium which includes: the harvesting of the producer cells, cell lysis procedures to release AAV vector, the clarification and removal of cellular impurities, vector separation and purification, and vector formulation and sterile filtration,” adds Chaudhary.
Most downstream approaches to viral vector separation and purification are based around traditional laboratory processes that are not scalable or suitable for clinical-grade manufacture because the viral vector field is still an emerging sector in biomanufacturing, Chaudhary also cautions. Despite the fact that the outcome of such conventional purification approaches would result in pure product, the lengthy processing time in manufacturing and the complexity of such a process results in a more expensive downstream purification procedure and ultimately, cumulative yield losses. “Therefore,” Chaudhary explains, “the lack of scalable platform technologies for purification has proven to be a bottleneck in downstream processing. In addition, it is important to maintain AAV vector biological activity when removing impurities and contaminates present in a feedstock that originates from host cells or culture media. Next to this, the vast majority of described AAV purification processes rely on the specific serotype produced. This requires the design of a new process for each different serotype.” Chaudhary further explains that the presence of empty capsids presents yet another challenge associated with AAV vector purification.
The gene therapy manufacturing process is an overall exhaustive and complex task, presenting manufacturing challenges such as the following:
- • Sterility and avoidance of adventitious agents. It is difficult to maintain a complete sterile environment using open systems that are extensively used in the manufacturing units.
- • Live viral vector. Gene therapies involving the use of a live viral vector need extra care during handling and manufacturing processes to maintain the potency of the virus. Improper handling leads to loss of viral potency.
- • Raw materials. Raw materials, such as growth factors and cytokines, that are required for manufacturing of gene therapy products are neither easily available nor affordable. In addition, the growth factors need to be continuously and manually added/replenished in the medium. This cumbersome process leads to an increase in the chances of errors.
- • Cell expansion environment. Growing a large number of cells is a challenging task due to lack of technologies to monitor the cell-expansion phase. Moreover, some of the cells are sensitive to chemical transfection reagents. Furthermore, because a cell-harvesting process involves several steps, the probability of committing mistakes is much higher.
- • Cell preservation. Freezing the cells for preservation is one of the major challenges as only a limited number of vessels are available for use in a cryopreservation environment, directly impacting the cell viability and quality.
“For certain manufacturing processes, downstream processing can be a bottleneck, for example, when ultracentrifugation is employed,” agrees Murphy. “Thermo Fisher has developed downstream processes using scalable technology (e.g., column chromatography) that can handle large volumes of intermediate while separating out impurities. This eliminates the downstream bottleneck often making upstream production the yield limiting step in the process,” he states. Another manufacturing challenge in viral vector production, Murphy points out, is the development and qualification of robust analytical methods to measure product quality.
“At the present time, there is currently a shortage of vector supply driven by the rapid growth of gene therapies both in vivo and ex vivo,” adds Vicaire. The shortage in viral vector supply is also under pressure from inefficient manufacturing process and the need for specific facilities with increased biosafety level (BSL-2), which are currently lacking, Vicaire points out.
The solution to increasing viral vector capacity involves both structural and technical issues. Vicaire states that a combination of structural evolution on the CDMO side and development of new technical solutions on equipment suppliers’ side can solve production challenges. “We must bring robust, scalable, industrialized manufacturing processes as fast as possible (less manual and more automated processes) and GMP solutions for both small-scale and large-scale production,” Vicaire says.
“The CDMO market is currently fragmented with more new players offering to manufacture vectors. However, the capacity is still not covering the current needs. Capacity increases should resolve the supply shortage, which will be followed by the consolidation of CMOs [contract manufacturing organizations] with smaller players being acquired,” Vicaire adds.
“On the technical side we must improve titer upstream (high titer stable producer cell line and/or cell line and media optimization) and for AAV specifically, improve ratio of full versus empty capsids; as well as improve downstream recovery (currently at best 30%, new nanofiber technologies coupled with next generation of ligands could significantly improve recovery),” Vicaire further explains.
At Cytiva, the company offers a structural solution to increasing viral vector manufacturing capacity through its KUBio box, a modular biomanufacturing environment. The company has tailored its KUBio product line to include a standardized bioproduction environment designed specifically for viral vector production. In addition, Cytiva has developed a technical solution that involves new fiber-based purification products (Fibro technology) that increases downstream recovery levels, according to Vicaire.
Exploring non-viral gene delivery vehicles
Viruses, however, are not the only type of delivery vehicle being explored for gene therapy. One area that is showing promise is with gene editing of cells that can then be re-administered to the patient to correct a genetic mutation, says Murphy. He highlights the fact that some innovators are using guide RNA with an endonuclease (e.g., Cas9) to modify cells ex vivo and make the edits. “I expect we’ll see some products approved in the future using this technology,” he asserts.
While there is a significant amount of work going into viral vectors to improve their tropism, rate of infection, and adverse immunological response, there is also much attention on developing other gene therapy mechanism options. “If we look beyond vector-based approaches, there are new CRISPR technologies that are moving into this space without some of the drawbacks of vector or virus-based systems,” Weiss adds. “Two of these approaches, Base editing and Prime editing, have the ability to introduce small changes or corrections to the host genome without introducing foreign DNA. Base editing has the potential to specifically change single nucleotides in a defined pattern (G–A, C–T, T–C, A–G) depending on the type of Cas9/deaminase complex being used. Prime editing, meanwhile, involves the use of a reverse transcriptase complexed to a Cas9 nickase in combination with an extended gRNA that contains an RNA template used to confer the desired edit,” Weiss explains. This process allows for a defined change to be made to a small region of the host genome without significant off-target and unintended mutations, he adds.
“Each of these CRISPR-based technologies open up new avenues to make targeted changes to a host genome with less of a potential immunologic response. There are certainly drawbacks with these approaches as well, and there is more work that needs to be done in this area, but it is a truly exciting development,” Weiss emphasizes.
“With gene therapies, both with episomal expression and gene integration, the main purpose is to have a long-lasting effect. At this moment viral vectors are the most promising technology. That being said, other technologies such mRNAs delivered to the target cells with, for example, mammalian cell-line produced engineered exosomes, start to show their capabilities,” Touw interjects. “As with all new technologies in biopharma, there are several hurdles to take, but we follow the developments in the field closely and take along the lessons learned from other molecules.”
“Plasmid vectors, meanwhile, are one of the most common non-viral gene delivery tools that are used to insert transgenes into target cells,” Chaudhary states. “Plasmids can be easily modified to deliver a therapeutic gene and optimize its expression in a host cell. The incorporation of a multiple cloning site into a plasmid enables the insertion of a gene of interest with the help of restriction enzymes. The circular DNA is nicked at the multiple cloning site and, after the incorporation of the gene of interest, the nicks are annealed via a ligation step. The gene present in these vectors is generally flanked by a promoter sequence and a transcription terminator sequence to facilitate proper expression after it is incorporated into the host genome. Plasmids with inducible promoters are widely preferred as gene delivery tools, owing to the fact that these vectors offer the flexibility to activate/deactivate gene expression as required,” Chaudhary explains.
Various modified versions of plasmid DNA vectors have been developed, including minicircles and minivectors, that are being used as vectors, Chaudhary further adds. “Apart from plasmids there are liposomes, lipoplexes, polyplexes, and oligonucleotides [that can be used] as non-viral modes of gene delivery.”
Vicaire also notes that using non-viral vehicles offers a safety benefit in that there is less immunotoxicity compared to viral vectors and potentially lower cost as well as ease of production, such as for synthetic or mechanical approaches. Challenges to using non-viral approaches, however, include poorer efficacy, the potential of shorter duration of the gene therapy expression (i.e., repeated dosing may be required), and poor targeted delivery because there may be less tissue specificity.
Reference
1. S. Lehrman, Nature 401, 517–518 (1999).