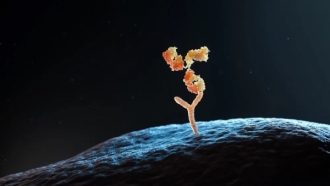
Manufacturing differences between traditional mAb therapies and newer biotherapeutics dictate whether processes should be scaled up, scaled out, or use an alternate approach for commercial production.
The approval of emerging biologic-based therapeutics—such as cell and gene therapies—presents challenges and decisions for drug companies looking to increase the manufacturing volumes of these specialized therapeutics. However, differences in manufacturing cycles for emerging therapies, compared with that of the traditional monoclonal antibody (mAb) therapeutic, calls for different strategies to increase production.
In mAb production, increasing production volume to commercial scale is typically a scaling operation. Bioreactor size can be increased (scale up), or the number of bioreactors used can be increased (scale out). In addition, mammalian cell culture technology has advanced such that producing multi-kilogram quantities of therapeutic mAbs in one batch is now commonplace (1). With a cell therapy, however, establishing cost-effective ways to commercially manufacture cell therapies for mainstream use remains a challenge. For a gene therapy, some aspects of its manufacturing process can use a scale-up strategy similar to a mAb, but other aspects of its manufacture cannot be easily be scaled up.
The cell therapy challenge
Cell therapies are generally divided into two categories: autologous and allogenic. Autologous cell therapies use a patient’s own cells for the threapy, while allogenic cell therapies use cells from a donor to develop a therapy to treat multiple patients. Specifically, autologous cell therapy manufacture is not easily scaled up; allogenic cell therapies have a better chance at following the traditional scale-up route.
With allogenic cell therapies, a single batch of donor cells is used to produce large volumes of the product for many patients, a model similar to traditional mAbs, in which a single cell-line source is cultivated to mass produce the product. The case for scaling up allogenic cell therapy production is thus supported by the economies of scale that apply to mAb production (i.e., scaling up production reduces the cost per dose of final therapeutic product) (2). In addition, both the engineering knowledge and operational expertise gleaned from scaling up mAb production already exists and can be used to support the increased manufacture of allogenic cell therapies, provided that a product quality profile for the allogenic cell therapy has been established and is well understood (2, 3). It is estimated that scale-up manufacturing solutions for cell therapies will need to generate batches of up to 2000 L (3) of product using single-use, disposable technology. Dependence on single-use formats limits the selection of upstream hardware that is currently available, however; downstream hardware are more limited because processing solutions that are currently available are typically incompatible with the unique requirements of cell-therapy products (4).
Yet, even if allogenic cell therapies lend themselves to a scale-up manufacturing strategy, there are limits to how scale up can be implemented for this type of therapy. Unlike mAb production—where the goal is to produce higher titers of cells containing the antibody—in cell-therapy, the cell itself is the therapeutic agent. The cells must, therefore, keep their integrity and remain functional, two aspects that are challenged when the cell density in the biomanufacturing environment increases. As the number of cells rise, the risk of introducing inhomogenity also increases; if inhomogeneity increases, then cellular performance can also be altered, impacting the quality and effectiveness of the cell therapy. By scaling up cell production, process developers and operators must also overcome the challenges of properly scaling other aspects of the manufacturing process, such as cell harvesting, downstream processing, and formulation technology, to ensure that the final cell therapy product meets quality standards and therapeutic requirements (2).
Autologous cell therapies, in comparison, do not lend themselves to a scale-up manufacturing strategy because each treatment is patient specific. Cells must first be collected from the patient, transported from patient to a manufacturing facility where the cells are processed, and then shipped back to the patient for administration. The entire manufacturing chain, from patient back to patient, must retain sterility and traceability. Thus, autologous cell therapies require a decentralized scaled-out manufacturing process—to produce a single batch of therapy for each patient. This poses a challenge to conserving cost making economies of scale difficult to achieve (3). Lowering the costs associated with commercial-scale autologous cell-therapy manufacture therefore relies on advanced engineering solutions and innovative manufacturing technologies that reduce the complexity and labor-intensive steps that currently exist in cell therapy manufacture (2).
For commercial autologous cell therapy production, a scale-out scenario would entail a complete manufacturing loop—from patient to manufacturing facility and back to patient—set up per patient. Challenges are largely rooted in the logistics of the workflow, such as the location of each patient, how close they are to a cell-therapy manufacturing facility, maintaining sterility and traceability throughout the entire loop, preserving cell integrity and functionality until the therapy is administered to the patient, and time constraints where cell viability is time dependent. Bioprocessing equipment and materials suppliers—as well as contract manufacturing organizations—are racing to develop suitable tools and processes to meet the manufacturing demands of these personalized treatments.
The gene therapy challenge
Gene therapy manufacturing has similarities to mAb manufacturing that allow for some steps in its process to be scaled up. Currently, viral vectors are a popular method used to deliver gene therapy. Mammalian cells are cultivated to produce the viral vector “package”, and—as in cell culturing for mAbs—cell density can be increased by using larger-volume bioreactors. In the case of gene therapy, you may scale out by adding more bioreactors. The downstream purification process can be scaled up, as the purification process for a gene therapy can use similar hardware as is used in mAb purification.
“In general, purification processes in gene therapy are able to be scaled up to larger volumes because most of them use standard types of biotechnology unit operations such as chromatography, membrane, and/or hollow fiber filtration. On the downstream purification side, much of the technologies one would use for gene therapy are commonly used on mAbs now,” says Diane Blumenthal, head, technical operations, Spark Therapeutics. Spark received FDA approval for its gene therapy product, Luxturna (voretigene neparvovec-rzyl), for treating an inherited retinal disease, in December 2017 (5).
“For example, mAbs rely heavily on affinity resins, which are very specific to the mAb itself. In the case of gene therapy, the resins would be specific to the capsid protein. The downstream purification aspect lends itself nicely to standard purification technology. Although there are many technical biochemical nuances to take into consideration, the unit operations themselves are very similar to mAbs,” Blumenthal adds.
For other steps of gene-therapy manufacturing, however, a scale-up strategy is more complex. One major challenge to a scale-up strategy lies in the crucial step of transient transfection, which is when DNA is introduced into the mammalian cell. The genetic material to be introduced into the mammalian cell include the DNA that encodes the cell’s machinery to make the virus, which will be the vehicle through which the therapy is delivered, and the DNA that will be delivered to the patient’s cells (i.e., the therapy). The virus packages the therapy DNA, making it ready for delivery (i.e., the viral vector).
The transient transfection process has not been perfected to be effective at significantly large volumes, and a significant barrier to that lies in the fact that the transfection process is a highly complex process, according to Blumenthal. There is a limitation on how much DNA each cell can take up. In addition, there are challenges posed by having high DNA densities present during transfection. DNA is sticky and especially likes to stick to itself and to cells. The more DNA there is in a mixture, the higher the chances that the transfection process will be less efficient.
“With a mAb, we can grow 10,000 L or more. But, [for a gene therapy] we haven’t quite perfected the ability to grow cells to a high cell density as we have been able to do with a mAb and still be able to conduct the transient transfection process. We haven’t perfected the technology to get us to those large volumes where we can produce gene therapy product in larger amounts, quickly, while improving upon the cost of goods economics,” Blumenthal states.
Based on the inherent limitations posed by the transient transfection process, a simple scale-up method may not work; a scale-out method may be more appropriate. That would entail making smaller cell culture batches and combining them together at an appropriate place in the production manufacturing process. “There needs to be a balance between how much DNA can be put into a concentrated volume, how high the cell density can be, and how capable the cell machinery is to take up the DNA and produce the required virus for packaging the therapeutic DNA component. Overall, it’s a very complex biological process. We understand the process, but we don’t yet understand well enough every little nuance nor the complex needs of the transient transfection process to be able to apply engineering principles to overcome the biochemistry, the virology, and the molecular biology that is associated with it,” Blumenthal says.
In the specific case of Luxturna, Spark uses a roller bottle process in the cell culture step, an older technology still used to manufacture some vaccines. In the roller bottle process, cells grow and attach to the surface of cylindrical bottles and are bathed in growth media as the bottle is rolled on a rack inside a temperature-controlled chamber. To increase production volumes using a roller bottle process, a scale-out strategy could be used. Scale-up technology is also available for this type of culture methodology. They are not, however, more efficient than suspension culture methodology.
“On the upstream cell-culture side, Luxturna is made using an attachment-dependent system which, when you really want to do more volume, you do multiple weeks’ worth of production at a certain scale and then we combine them together at the final step, after the product has been purified,” Blumenthal elaborates.
Spark is, however, looking toward advancements in technology for their processes. For example, the company is moving almost all of its products to more standardized technology. In the case of the cell culture process, it is moving toward a suspension cell culture (i.e., bioreactor), so it would no longer need to rely on an attachment-dependent cell culture. “Using a bioreactor allows for us to scale up as well as scale out,” Blumenthal says.
References
1. S. Aldington and J. Bonnerjea, Journal of Chromatography B 848 (1) 64–78 (2007).
2. T. Heathman, “Scalability in Cell-based Therapy Manufacture,” TechnologyNetworks.com, Feb. 26, 2016.
3. M. Varley and M. Brady, “To Scale-Up or Scale-Out? The Challenge of Commercializing Cell Therapies,” Cambridge-Design.com, July 11, 2017.
4. G.M. Pigeau, E. Csaszar, and A. Dulgar-Tulloch, Front. Med. 5 (233) (2018).
5. FDA, “FDA Approves Novel Gene Therapy to Treat Patients with a Rare Form of Inherited Vision Loss,” Press Release, Dec. 18, 2017.